Bacterial translocation in sepsis and acute respiratory distress syndrome (ARDS)
Some notes on gut dysbiosis in SARS-COV2, and possible evidence of gut microbe involvement in sepsis.
Originally this post was going to dive into the bombastic phrase of “pathobiont to pathogenic1”, as noted in the previous article’s rather sensationalist concluding remarks.
The phrase “pathobiont to pathogenic” refers to situations in which many commensal bacteria (bacteria that exist as part of our microbiome) may utilize opportunities to infect the host, shifting over from rather benign entities into those that may cause disease. I should also point out that the phrase is absolutely not original, as I noticed it one of the articles I was reading, although I can’t recall which one contained this phrase…
Such cases of “p2p” may include viral infections, poor nutrition, and chronic disease such as inflammation; all situations in which the microbiome may be damaged and lead to dysbiosis. It is the dysbiosis that tends to correlate with opportunistic infections, as it provides an opportunity for select bacteria to colonize and bloom.
However, after reviewing some of the literature with respect to viral infections (the topic in the prior post), I unfortunately found that much of the information is rather limited.
Many studies citing secondary bacterial infection may not mention where the bacteria came from. Instead, many papers report the observation of various bacterial strains, but many do not confirm whether the bacteria were of community (i.e. environmental) origin or from the host’s own bacteria.
For instance, studies may note changes to the gut flora of people suffering various diseases such as cirrhosis, HIV, cancer, and infections such as Influenza. They may also note the increased risk of a bacterial infection. However, they tend not to indicate whether the change in microbiome may elevate opportunistic bacteria, and whether it’s these strains of bacteria that are related to the secondary infection.
So there tends to be a lack of a linking feature. It’s likely that many of these studies are rather novel in their findings and may take several years until additional information comes out that helps better piece together the commensal bacteria’s role in secondary infection.
Or I may not be looking in the right places. 🤷♂️ But unfortunately, I apologize as some of my initial endeavors have come up a bit fruitless in my search for a salacious tagline.
*With that, we’ll take a brief look at SARS-COV2 and dysbiosis, as well as an interesting study noting a possible relationship between the translocation of gut microbes in incidences of sepsis and ARDS.
SARS-COV2 and related dysbiosis
Now, even with that being said I came across two rather fascinating studies that appear to provide some insights into sepsis and even possibly some mechanisms of ARDS as it relates to SARS-COV2.
But before diving into these studies it’s worth taking another look at SARS-COV2 and gut dysbiosis.
It’s know that SARS-COV2, similar to other viral infections, may alter the microbiome of those infected.
For instance, Harper, et al.2 notes several studies detailing some of the altered gut microbes, including some which may be considered as opportunistic bacteria [context added]:
These observations [positive stool samples and GI issues in SARS-COV2 infected individuals] raise the possibility of the GI microbiome as a variable in the disease. In fact, an early limited case series of COVID-19 patients in China found reduced members of the genus Lactobacillus (sensu lato) and Bifidobacterium (Xu et al., 2020). Two subsequent studies comparing COVID-19 patients with both healthy controls and hospitalized patients with other respiratory infections, have found significant disturbances in the gut microbiota of COVID-19 patients (Zuo et al., 2020; Gu et al., 2020). More specifically, opportunistic pathogens like Actinomyces, Erysipelaclostridium, Streptococcus, Veillonella, Rothia, and Enterobacter were associated with COVID-19 diagnosis and/or severity among studies.
Note that the species of bacteria aren’t necessary to remember, but that many beneficial bacteria were considered to have been low in population while opportunistic ones were relatively high.
Interestingly, the study from Zuo, et al3, which looked at 15 individuals hospitalized with SARS-COV2 (7 antibiotic naïve and 8 given antibiotics), found a correlation between a few strains of bacteria associated with bacteremia (bacteria in the blood) and one associated with ACEII expression correlating with COVID severity:
Three bacterial members from the Firmicutes phylum, the genus Coprobacillus, the species Clostridium ramosum and C. hathewayi, were the top bacteria positively associated with COVID-19 disease severity (Spearman correlation coefficient Rho >0.9, P < .01, Table 3). Both C. ramosum and C. hathewayi have been associated with human infection and bacteremia.27 , 29 Importantly, Coprobacillus bacterium has been shown to strongly upregulate colonic expression of ACE2 in the murine gut.21
In contrast, bacteria associated with reduced gut ACEII expression (four Bacteroides species from the study), gut homeostasis maintenance (Alistipes onderdonkii), and anti-inflammation (Faecalibacterium prausnitzii) were negatively correlated with COVID severity.
The overall findings of the study can be summarized in the figure below with the right box noting the alterations and possible protective bacteria.
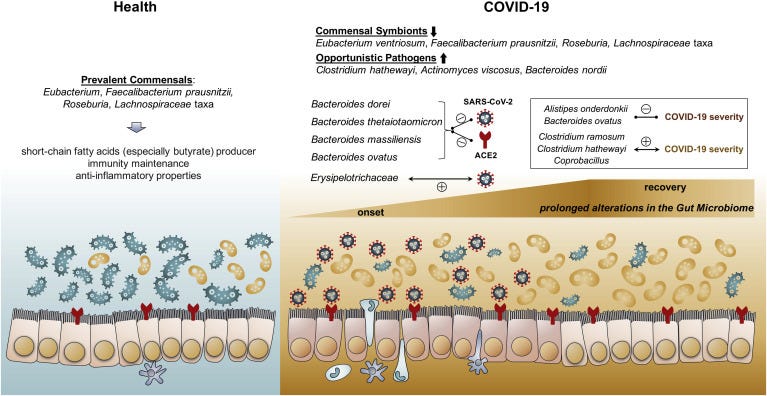
It’s important to remember that microbiome studies are heavily confounded by the use of antibiotics and the timing of sample collection. Antibiotics will have an inherent influence on the gut microbiome, and the inability to reference individual microbiome profiles of patients prior to infection runs into the conundrum of from vs with i.e. is the gut makeup from the infection or due to other circumstances.
This differentiation is important, as it may provide predictive value in certain bacteria species, such that some bacteria may help reduce COVID severity and be protective against infection.
In the case of the Zuo, et al. study 15 healthy patients were used as a reference, which adds some strength to the results but still runs into issues of lacking individual baseline measures and may suffer from heterogeneity bias.
In contrast, the results associating beneficial bacteria to COVID severity may at least allude to a protective role of some of these bacteria due to downregulation of ACEII, although some reports have suggested that SARS-COV2 may downregulate ACEII expression for its own benefit, adding additional complexity to the situation.4
And so evidence continues to provide insights into the microbiome during a SARS-COV2 infection, and general consensus appears to indicate an abrupt change to the gut microbiome.
Now, here is where we may note a possible case of “pathobiont to pathogen”, as some evidence suggests that gut microbes may migrate to the lungs, and may be associated with pathogenesis of sepsis and ARDS. Given that severe cases of SARS-COV2 may lead to ARDS, it may be worth considering the relationship between microbe translocation and ARDS in COVID patients.
Microbe Translocation in Sepsis and ARDS
Our body is comprised of many different microbiomes where bacteria of all sorts reside. Our lungs5, reproductive organs, urinary tract, skin, nose, mouth, and intestines all play host to commensal bacteria. In fact, we may even find that there may be a special place in our hearts (both literally and figuratively) for beneficial bacteria.
However, as many organs and tissues are made up of their own microenvironment with different acidities, oxygen levels, and nutrients it wouldn’t be far-fetched to assume that distinct bacteria may occupy different environments and spend most of their time within their microenvironment.
But under certain circumstances the bacteria may move, and when they take host in different organs and tissues they may actually become pathogenic.
This movement of bacteria is generally referred to as bacterial translocation, although some researchers may also refer to this as bacterial migration. A broader term, microbial translocation, may also be used which includes products from microbes such as antigens and lipopolysaccharides that move outside of their respective microenvironments into the neighboring environment and tissues.
Regardless of the term, evidence generally points to bacterial translocation as being associated with many diseases.
Although outside the scope of this review, here are a few diseases with evidence of bacterial translocation for those interested. Note that I have not looked deeply into these articles so a word of caution as to the actual content:
Gómez-Hurtado, et al.6: Microbiome and bacterial translocation in cirrhosis
This article is unfortunately in Spanish so I couldn’t assess the information. Maybe those that know Spanish can read the review and examine it for themselves.
Zevin, et al.7: Microbial translocation and microbiome dysbiosis in HIV-associated immune activation.
Kouzu, et al.8: Bacterial Translocation in Gastrointestinal Cancers and Cancer Treatment.
With cancer and other invasive interventions it’s important to consider that bacterial translocation may be due to the procedure/intervention and not necessarily the cancer alone. So there’s a few variables worth considering within this context, although it’s also suggested that bacterial translocation and the inflammatory response may be related to carcinogenesis.
With that, the pertinent article will be one from Dickson, et al.9, which examined a movement of gut bacteria to the lung in patients with sepsis and ARDS, suggesting a possible role of this bacterial migration in the pathogenesis of these diseases.
Although a relatively short study, there’s several moving pieces that may make it a bit confusing, including the use of many acronyms and abbreviations. To help with the study a few words and phrases will be clarified.
Relevant Terminology
caecal ligation and puncture (CLP): CLP is considered to be a “golden standard” of sepsis modeling in mice. Generally, the junction between the small and large intestines of mice are ligated (tied into a knot), causing necrosis of the intestinal tissue. This is also paired with perforation of the cecum itself, which allows fecal material to spill into the peritoneal (abdominal) cavity. Both actions eventually lead to sepsis of the animals. It’s been argued that CLP provides a window into the progression of sepsis that is comparable to humans, however some criticisms have come about due to a few studies not being able to translate mice model findings into the clinical setting (Dejager, et al.10).
Sepsis: Sepsis is a hyperinflammatory response towards an insult such as a bacterium, virus, or injury. However, this hyperinflammatory response may lead to damage to tissues and organs, causing serious life-threatening issues to the individual.
Acute Respiratory Distress Syndrome (ARDS): Although a familiar term through COVID it may be worth defining. ARDS is an acute event characterized by lung injury (both endothelial and alveolar), diffuse inflammation, and reduced oxygen capacity. It’s been suggested that sepsis is one of the main causes of ARDS.11
Branchoalveolar Lavage (BAL): A minimally invasive procedure in which saline solution is administered through a sterile implement into a subsegment of the lung and vacuumed back up. This method is used to collect samples from the lungs. In this study BAL will be referenced continuously, and just refers to the samples collected from patients with ARDS in order to sequence the microbes in their lungs.12
Operational Taxonomic Unit (OTU): OTUs are used to classify bacteria based on genomic sequence similarities. Because the microbiome and virome have not been fully elucidated, bacteria and viruses may be differentiated based on OTU numbers in cases where they cannot be identified by typical means. It’s not important to know the OTU number, but if it appears often in the study, as in the phrase “Bacteroides OTU” it refers to species of bacteria which share genomic similarities to the Bacteroides genus. In particular, the study references Bacteriodales sp. OTU008, which encapsulates bacteria that fall under the Bacteriodales order. Note that the mice study use the designation of OTU008 while the human studies used OTU009. When looking at the information below remember that the human trial looked at bacteria from the Bacteroides genus (OTU009) which came from the same Bacteriodales order in the mice studies.
For additional context, the terms order, phylum, genus, and species may be thrown around in the proceeding text. Note that these refer to taxonomy hierarchies for organisms, starting from the broadest domain all the way down to species-specific. In high school we were taught the hierarchy with the following (from broadest to most specific):
Dumb Kings Play Chess On Fine Green Sand
Domain → Kingdom → Phylum →Class → Order → Family → Genus → Species
As mentioned in previous posts, taxonomy can get really confusing when categories are named very similarly. For instance, Bacteroidetes are a phylum, Bacteroidales are an order, and Bacteroides may refer to a genus. Altogether, they all can get super confusing, and I may even mix them up here as well! So just be aware that if these classifications appear below they are noted different taxonomy hierarchies.
Now, as mentioned the study used mice as a model of sepsis. However, we will skim over this portion citing only the key findings.
Key Findings in Mice
At 24 hours post-experimental sepsis via CLP the lung microbiome of mice began to alter, showing higher richness (detectable bacteria) for two bacteria from the order Bacteriodales in particular, relative to untreated mice and mice given a sham surgery (Fig. 1). Bacteria belonging to the Bacteriodales order are some of the densest colonies within the gut. Note that these bacteria were unidentified, and so were designated with the OTU classification, as well as the name Bacteriodales sp.
In another experiment with extended timepoints post-septic mice (CLP mice) showed distinct lung diversity relative to the untreated group and the sham group at 5 days post-intervention (Fig. 2a). However, the lung diversity in post-septic mice began to reflect control mice by 2 weeks post-intervention (Fig. 2b), suggesting that the lung microbiota may begin to normalize after several weeks.
It’s important to note that the mice within this experiment were given an antibiotic named imipenem, with a 2021 study suggesting better outcomes in post-septic mice given imipenem when liver pathology was analyzed13. In that regard, this normalization effect likely reflects antibiotic treatment, as the lung diversity of post-septic mice at 2 and 8 weeks reflected the lung diversity of imipenem-control mice. The authors note that the lung diversity in untreated (no antibiotic or sepsis) mice and 8 week post-sepsis mice were indistinguishable, however the spread of diversity appears more similar to the spread of imipenem-control mice at 5 days.
Lastly, researchers wanted to see where the Bacteroidales came from. When comparing the microbes found in the oral cavity of mice to those found in the gut for Bacteroidales, the researchers noted that these bacteria appear to only be found in the gut (Fig 3b).
Researchers also noted culturable Enterococcus, the second-highest bacteria found in the gut (Fig 3c) as another gut bacteria found in post-sepsis mice. The culturing of Enterococcus served as a proxy for viable Bacteriodales sp., as Enterococcus is an aerobic bacteria. Remember that sequencing sequencing doesn’t provide any measure of living bacteria. Therefore, the elevated levels of Enterococcus within the lungs of post-sepsis mice relative to controls suggests possible translocation of this bacteria, and thus possible translocation of Bacteriodales sp.
Interestingly, when researchers conducted direct lung injury via lipopolysaccharides (LPS), none of the post-sepsis Bacteroidales were noticed within the lung, suggesting that the movement of Bacteroidales to the lung was due to indirect lung damage via sepsis and translocation from the gut (Fig 3c), rather than direct injury to the lung itself.
Overall, these key findings in mice suggest a possible model in which microbes may translocate from the gut to the lungs via sepsis. What’s worth noting is that many Bacteroides are obligate anaerobes, meaning that they grow in places that lack oxygen. The fact that bacteria that thrive in low oxygen environment appear in the lungs during sepsis suggests physiological changes that reduce oxygen levels, allowing these bacteria to thrive in this changing environment. In the case of these murine models it’s hard to parse the actual nature of these bacteria (anaerobic vs aerobic), but this will be examined in the clinical models of ARDS.
It’s also important to note that the researchers are unaware of how the microbes moved from the gut to the lungs, but were only able to detect the presence of the bacteria.
It’s been suggested in a review from Wexler, H. M.14 that increased gut permeability may be play a role in the translocation of the microbiomes, which may find an easier route into the bloodstream. However, the use of CLP creates direct access of bacteria to the bloodstream, and so may only be reflective of punctured intestines in humans rather than sepsis caused by viral or bacterial infection.
And so although the mouse model suggests a possible mode of sepsis and gut microbe translocation, it doesn’t provide a model of infection leading to sepsis formation. It also doesn’t explain what changes to the lungs may allow for anaerobic bacteria to take hold.
Keep this in mind while we examine the human cases of ARDS and suggested gut translocation.
Clinical Key Findings
Lung microbiome of ARDS patients were enriched with uncultured gut microbes
Following the results of the murine models the researchers looked at 100 BAL samples taken from 68 patients exhibiting ARDS, looking for the presence of related Bacteriodales sp. as noted in the post-septic mice.
Interestingly, BAL samples were not able to culture these bacteria, although molecular assessments were able to identify the unknown Bacteriodales sp. from the murine studies as four anaerobic gut species (emphasis mine):
We employed analysis of multivariate abundance (mvabund) to identify taxa enriched in BAL specimens from patients with ARDS relative to healthy subjects (Supplementary Fig. 3b); of these, we focused on a prominent member of the Bacteroides genus (OTU009) that was classified to the same order (Bacteroidales) as the OTUs enriched in the post-sepsis lungs in our murine experiments. Alignment searches of databases revealed perfect alignment (100% coverage, 100% alignment) between this Bacteroides sp. and four anaerobic species abundant in the human gut20–22: B. fragilis, B. thetaiotaomicron, B. faecichinchillae and B. salyersiae (Supplementary Fig. 4). Of the 24 BAL specimens in this cohort studied by aerobic culture at the University of Michigan Clinical Microbiology Laboratory, none grew Bacteroides sp., despite culture-independent identification of this organism in eight (33%), confirming the limited sensitivity of aerobic culture-based techniques in identifying gut-associated anaerobes in respiratory specimens.
So here the researchers were able to identify the previously unelucidated Bacteriodales sp. found within the murine post-sepsis models as being anaerobic gut bacteria in humans. This was confirmed by the inability to culture these bacteria, as typical culturing methods rely on aerobic cultures and therefore would not be conducive to anaerobes.
Of note, B. fragilis has been implicated for its pathogenicity in many diseases, so the presence of B fragilis as one of the possible species is rather interesting. Also, all 4 of the bacteria are anaerobic, and therefore may reflect the opportunistic role of these bacteria in colonizing the damaged lung microenvironment.
It’s also worth noting that not all BAL samples contained these Bacteriodes OTUs, as only 33% of the BAL samples had detectable levels of Bacteroides OTUs. These BAL samples apparently represented 41% of the patients in this study, suggesting a bias in sampling as a few patients may have had several BAL samples collected.
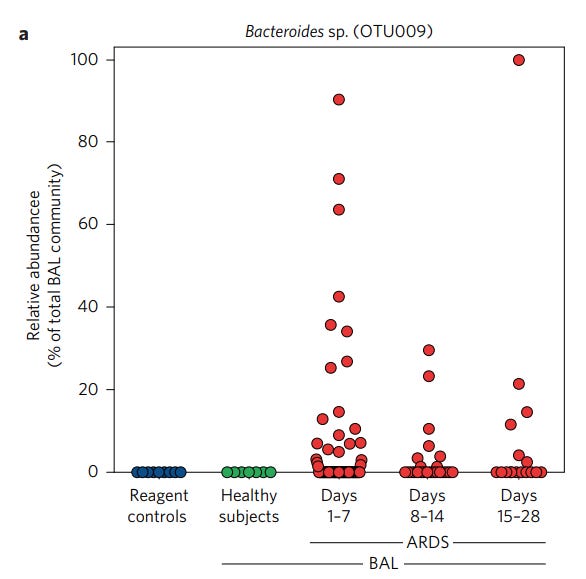
Altogether, the examination of humans with ARDS found similar results as the murine models, in that several anaerobic gut bacteria were located in the lungs of post-sepsis mice as well as ARDS patients.
In summary, these data demonstrate that the lung microbiome is enriched with gut bacteria in experimental sepsis and human ARDS. Enrichment with gut bacteria is significantly associated with key features of systemic inflammation. Alveolar TNF-α, a key mediator of the alveolar inflammation of ARDS, is significantly correlated with disorder of the lung microbiome. Our findings suggest that gut–lung translocation and alteration of the lung microbiome may represent a common mechanism of pathogenesis in sepsis and ARDS, and suggest potential therapeutic targets for the prevention and treatment of these common and lethal diseases.
It’s important to remember that this study is more of an observation, as the researchers did not get baseline lung measures of ARDS patients. Although the presence of these bacteria were elevated in various ARDS patients, their presence may also precede the incidents of ARDS.
Also, the patients included in this study were ones recruited for a clinical trial of a therapeutic intended to induce alveolar macrophages, and so this may have influenced the results in this study as well.
Patients were collected from 3 different hospitals, and it was noted that all 18 of the patients from the University of Michigan (representing 24 of the BAL samples) were given antibiotics at the point of hospital admission, with several patients being administered multiple antibiotics:
Additional information including clinical microbiology results and antibiotic exposure was obtained from the electronic medical records of 18 subjects enrolled at the University of Michigan Medical Center, representing 24 total BAL fluid specimens. Of these, at the time of bronchoscopy, all specimens (100%) were obtained while the subject was receiving antibiotics and most (83.3%) were obtained while subjects were receiving multiple antibiotics. The most common antibiotics used were vancomycin (50%), piperacillin-tazobactam (41.7%), levofloxacin (41.7%) and cefepime (16.7%).
And probably one of the most critical limitations of this study, a majority of the patients included did not have sepsis:
The most common ARDS risk factors were pneumonia (29.4%), sepsis (26.4%) and aspiration (20.6%). Other aetiologies included pancreatitis, trauma and transfusion-related lung injury.
Given the combination of antibiotic use and limited sepsis data, it would be critical to see how many sepsis and pneumonia patients had detectable Bacteroides OTUs within the initial 7 days of ARDS onset.
Key Takeaways
Well, you’ve reached this far into the laborious article and may be wondering what the point of this article was.
This article was a pivot to something I was investigating before due to lack of information (sorry readers!), so this was an alteration of perspective.
But aside from that, the key takeaway from this post is to highlight the role of the microbiome in sepsis and ARDS. As noted by Dickson, et. al. it’s been assumed that the microbiome plays some role in sepsis, but evidence hasn’t yet provided a mechanism for this role:
Numerous experimental and clinical observations over decades of study have demonstrated that the gut microbiome plays a key role in the pathogenesis of sepsis and ARDS5 : antibiotic-suppressed and germ-free animals are protected from the lung injury and mortality of experimental sepsis6–8 and numerous clinical trials have demonstrated that prophylactic suppression of gut microbiota with antibiotics is protective against multiorgan failure and mortality in patients with critical illness5,9. Yet the mechanism of the gut microbiome’s role in sepsis and ARDS is undetermined5,10,11 and previous studies have been limited by a dependence on culture-based techniques of microbial identification12.
The current study suggests a role in which the gut microbiome may move from the gut to other regions of the body, in this case the lungs, and this movement may contribute to pathogenesis.
Given this finding, it provides some perspective towards microbe movement in other diseases, and it could be that several cases of sepsis and ARDS may depend on the movement of bacteria and the induction of inflammation.
It’s quite possible that sepsis and ARDS may be one prime example of “pathobiont/commensal to pathogenic”.
Dickson, et al. hasn’t properly addressed the event of sepsis. However, we’ve previously documented the role of viral infection in secondary bacterial co-infections.
The inflammation, change in nutrition and metabolism, and direct damage from a pathogen all may contribute to changes in the gut microbiome, which may lead to sepsis and ARDS from bacterial translocation.
When we consider that severe COVID may progress into ARDS, and that COVID has been associated with dysbiosis, then it may also be worth considering what role bacterial translocation due to SARS-COV2 and dysbiosis may play in ARDS.
To that, the next article will look at a recent study noting gut bacteria in the blood of severe SARS-COV2 patients.
If you enjoyed this post and other works please consider supporting me through a paid Substack subscription or through my Ko-fi. Any bit helps, and it encourages independent creators and journalists outside the mainstream.

Note that the use of pathobiont here may be improper. It may be more appropriate to use a broader term such as commensal as these bacteria are a part of us irrespective of their ability to become pathogenic.
Harper, A., Vijayakumar, V., Ouwehand, A. C., Ter Haar, J., Obis, D., Espadaler, J., Binda, S., Desiraju, S., & Day, R. (2021). Viral Infections, the Microbiome, and Probiotics. Frontiers in cellular and infection microbiology, 10, 596166. https://doi.org/10.3389/fcimb.2020.596166
Zuo, T., Zhang, F., Lui, G. C. Y., Yeoh, Y. K., Li, A. Y. L., Zhan, H., Wan, Y., Chung, A. C. K., Cheung, C. P., Chen, N., Lai, C. K. C., Chen, Z., Tso, E. Y. K., Fung, K. S. C., Chan, V., Ling, L., Joynt, G., Hui, D. S. C., Chan, F. K. L., Chan, P. K. S., … Ng, S. C. (2020). Alterations in Gut Microbiota of Patients With COVID-19 During Time of Hospitalization. Gastroenterology, 159(3), 944–955.e8. https://doi.org/10.1053/j.gastro.2020.05.048
It’s quite possible that it’s not the downregulation of ACEII that’s critical, but how the ACEII is being downregulated. The event of downregulation from viral infection may come with far more damaging effects as compared to commensal bacteria regulation of ACEII expression, which may be more controlled and also regulated by a healthy microbiome.
Strangely, even though the lungs have a direct route to the environment through our nose and mouth, it’s only within the past few years that the lungs have been considered to have their own microbiome. Prior to this, it’s been assumed that the lung environment was actually sterile. It’s another indication of how science isn’t as far ahead as we may believe.
A comprehensive review of the lung microbiome can be seen in the following review. I haven’t been able to look through it fully, but it appears to be full of information. Also, you may notice that one of the co-authors of the review is the primary author of the study in question in this post:
Huffnagle, G., Dickson, R. & Lukacs, N. The respiratory tract microbiome and lung inflammation: a two-way street. Mucosal Immunol 10, 299–306 (2017). https://doi.org/10.1038/mi.2016.108
Gómez-Hurtado, I., Such, J., & Francés, R. (2016). Microbiome and bacterial translocation in cirrhosis. Microbioma y traslocación bacteriana en la cirrosis. Gastroenterologia y hepatologia, 39(10), 687–696. https://doi.org/10.1016/j.gastrohep.2015.10.013
Zevin, A. S., McKinnon, L., Burgener, A., & Klatt, N. R. (2016). Microbial translocation and microbiome dysbiosis in HIV-associated immune activation. Current opinion in HIV and AIDS, 11(2), 182–190. https://doi.org/10.1097/COH.0000000000000234
Kouzu, K., Tsujimoto, H., Kishi, Y., Ueno, H., & Shinomiya, N. (2022). Bacterial Translocation in Gastrointestinal Cancers and Cancer Treatment. Biomedicines, 10(2), 380. https://doi.org/10.3390/biomedicines10020380
Dickson, R. P., Singer, B. H., Newstead, M. W., Falkowski, N. R., Erb-Downward, J. R., Standiford, T. J., & Huffnagle, G. B. (2016). Enrichment of the lung microbiome with gut bacteria in sepsis and the acute respiratory distress syndrome. Nature microbiology, 1(10), 16113. https://doi.org/10.1038/nmicrobiol.2016.113
Dejager, L., Pinheiro, I., Dejonckheere, E., & Libert, C. (2011). Cecal ligation and puncture: the gold standard model for polymicrobial sepsis?. Trends in microbiology, 19(4), 198–208. https://doi.org/10.1016/j.tim.2011.01.001
Diamond M, Peniston HL, Sanghavi D, et al. Acute Respiratory Distress Syndrome. [Updated 2022 Oct 30]. In: StatPearls [Internet]. Treasure Island (FL): StatPearls Publishing; 2022 Jan-. Available from: https://www.ncbi.nlm.nih.gov/books/NBK436002/
Patel PH, Antoine M, Ullah S. Bronchoalveolar Lavage. [Updated 2022 Aug 28]. In: StatPearls [Internet]. Treasure Island (FL): StatPearls Publishing; 2022 Jan-. Available from: https://www.ncbi.nlm.nih.gov/books/NBK430762/
Khosrojerdi, A., Soudi, S., Zavaran Hosseini, A., Ghaffari Khaligh, S., & Hashemi, S. M. (2021). Imipenem alters systemic and liver inflammatory responses in CLP- induced sepsis mice in a dose-dependent manner. International immunopharmacology, 93, 107421. https://doi.org/10.1016/j.intimp.2021.107421
Wexler H. M. (2007). Bacteroides: the good, the bad, and the nitty-gritty. Clinical microbiology reviews, 20(4), 593–621. https://doi.org/10.1128/CMR.00008-07
This is an important part of the Covid-19 puzzle, that affects long haulers, who are stuck with a bewildering array of somewhat conflicting symptoms, from loss of smell an taste, tinnitus, headache, vascular and endothelial impairments, fatigue, cachexia, loss of appetite, weight, and dysbiosis. How are they all connected? Stress!
Dr. Bruce Patterson found monocytes infected with Sars-Cov-2 or it’s proteins. Dr.Juan Mauricio and his team found that “sun-threshold stress was enough to recruit monocytes from the spleen to the brain, where they shape-shift in macrophages that toggle M1 anti inflammation with fractalkine and M2 inflammation. With fibrin. If these macrophages are infected they will release fibrin and fractalkine which is a neurotoxin and adhesion molecule that explains headaches and loss of memory and taste, and it also explains why blood thinners do not break up blood clots. Here’s the thing, our guts do the same thing as M1:M2 macrophages in controlling inflammation of our microbiome. Ask yourself, how do bats have Ebola. Coronavirus and rabies in their microbiome? Then ask yourself how do bats live the longest of all mammals for their size, 230 human years and hardly any cancer? GI hormesis. Remember the king who defied the Romans by ingesting poison to prevent being poisoned? Well that is what your gut does. If invaded by a virus, it will produce inflammatory Th17 cells, but these will be matched by twice as many anti inflammatory T-Reg cells, so long as you have enough segmented filamentous bacteria like B. Fragilis that we no longer have. Liminal stress, below the level of awareness is controlled from the thalamus for speed of reaction to infection, which is a good this, but if macrophages are infected and disseminated all over the body, and we have few commensals, is gut diversity to offset disease, the we are in trouble. Dr. Bruce Patterson of the Covid Chronic care, uses statins to block fractalkine and antivirals to attack the viral reservoirs, buts it’s up to us to control stress inside of us and social stress of out industrial society, and crest biodiversity so our children can have a Noah’s Arc microbiome.
Gut tissues can retain SARS-CoV-2 particles after COVID-19 infection for more than one year after the resolution of COVID-19
Evinco Health
https://www.linkedin.com/posts/rachel-jessey_health-environmental-freshair-activity-7016743013699715072-TisK
Lung Health and the lung microbiome
Interesting concepts discussed here.
I have read through this interesting review and although the sole focus is not on SARS-CoV-2 infection there are some interesting concepts discussed in relation to the human lung microbiome and disease.
The authors conclude that there is a bi-directional relationship between microbial ecosystems and host susceptibility to infectious and other diseases.
1) Microbiomes may serve as the source of infection-causing pathogens
2) Invading pathogens have the ability to alter the host's microbial communities for extended periods of time.
Also discussed are the environmental insults that predispose not only hosts' tissues but also human microbiota to the disease development by changing microbial composition, host immunity, and epithelial integrity.
What was really interesting to me as a promoter of simple yet effective interventions is that there is mention of replenishing and promoting certain species from exposure to rich and healthy ambient air microbiomes (e.g. forest microbiome).
These are important concepts to consider when trying to understand the relationship between our environmental inputs, our microbiome interactions and health.
Human airway and lung microbiome at the crossroad of health and disease
https://www.spandidos-publications.com//10.3892/etm.2022.11718