Tuesday’s post provided a glimpse into the possible future of NAC, with a slight forewarning of how the FDA may categorize NAC or allow for its availability in the future.
I saw a few people comment that they decided to order additional NAC in case the ruling leads to the removal of NAC from shelves.
As a heads up, remember that the information I provide here is not medical advice.
But more importantly, I’m always concerned that people are taking compounds (from both sides) without actually knowing the rationale for a drug’s use, or what effects it may have on the body. Instead, it feels like people are sort of told to take something with a sentence or two noting a little bit about the supplement without explaining how that supplement works.
How does Vitamin D boost the immune system? Why does NAC have antioxidant properties?
It’s why I generally include pharmacological information on drugs and compounds, mostly to show people that they need to have an understanding of what they put inside their body, rather than just doing it because other people say it’s good for them.
But that’s enough of a rant. This post will follow along with yesterday’s remarks on NAC and provide some of that pharmacological data so that you all can become informed as to what NAC may do inside of our bodies.
General Background
Most other compounds we have reviewed before have generally been complex in structure. For example, Quercetin, Nirmatrelvir, Ivermectin, and Molnupiravir can be seen below, comprised of several functional groups and a few are rather bulky molecules. I won’t tell you which is which- refer to notes here or elsewhere in order to figure it out 😉.
Aside from Zinc, NAC probably has one of the most simple molecular structures within the COVID supplement arsenal.
NAC is comprised of an L-cysteine1 amino acid with an acetyl group attached to the amine.
I’ve tried to find natural sources of NAC, but many articles refer specifically to foods rich in L-cysteine such as meat and eggs and not specifically to NAC.
Surprisingly, it appears that NAC is actually synthetic and not found in nature.
This may make sense as to why it is categorized as a drug, as its first discovery may have come from clinical trials looking for possible therapeutics, and it is likely here where synthesis of NAC first came about.
The first uses of NAC occurred in the 1960s when it was first considered a mucolytic (breaks up mucous) agent (Perde, et. al.2):
The NAC story started in earnest in the early 1960s when scientists at Mead Johnson recognized that NAC is an effective agent for the liquefaction of mucoid secretions. The first clinical study confirmed it to clear the tracheobronchial airway in patients suffering from a range of acute and chronic obstructive lung diseases (Webb, 1962). On May 28, 1963, the US Patent Office granted patent 3.091.569 for “Mucolytic N-Acylated Sulfhydryl Compositions and Process for Treating Animal Mucus” to Mead Johnson & Company, Evansville, Indiana.
Then in the 1970s studies showed that NAC was hepatoprotective against Acetaminophen overdose:
Another major chapter of the NAC story unfolded in the 1970s when intravenous NAC was established to protect against severe liver damage caused by paracetamol (acetaminophen) poisoning. Following the demonstration of its protective effects in animals (Piperno & Berssenbruegge, 1976), clinical studies confirmed the efficacy of NAC in the treatment of paracetamol poisoning. Intravenous NAC, supplied within 10 hours of paracetamol ingestion, at a dose of 300 mg/kg (given over 20 hours) prevented liver damage, renal failure, and death in patients with severe paracetamol poisoning (Prescott, Park, Ballantyne, Adriaenssens, & Proudfoot, 1977).
Eventually NAC would be marketed as a dietary supplement, leading to the current predicament we are experiencing.
NAC can take on multiple active forms including NAC itself, free L-cysteine after NAC undergoes deacetylation, and the tripeptide glutathione which contains L-cysteine as the central amino acid. It’s assumed that each form elicits its own therapeutic actions, and we’ll discuss some of these further on.
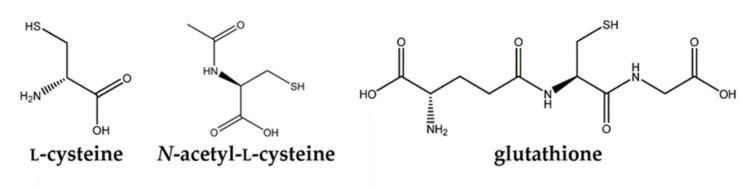
Pharmacokinetics
NAC is available in several forms including intravenous (IV), nebulized, and oral via supplementation.
The oral form of NAC is the least bioavailable, suffering from the first pass effect and early metabolism before reaching necessary organs and cells (Tenório, et. al.3):
The bioavailability of free NAC is very low (<10%), and only a tiny amount of the intact molecule reaches the plasma and tissues [13,14]. Additionally, due to the variety of ways that NAC can be found in plasma (oxidized, reduced, and bound to proteins), its pharmacokinetics (PK) is not yet fully understood [15,16]. NAC can be oxidized to a disulfide, N,N′-diacetylcystine, and it still generates mixed disulfides via a reaction with other low-molecular-weight thiols. After their complete metabolism, cysteine, cystine, inorganic sulfate, and glutathione are the primary metabolic products produced [14].
Because of the first pass effect, NAC is considered more effective when given intravenously rather than orally.
There are also a few problems with oral administration of NAC. As a capsule, NAC may undergo oxidation to form disulfide bridges (NAC-S-S-NAC) between NAC monomers.
Here’s a perspective from Herzenberg, L. A.4 who was one of the first researchers to look into NAC as a therapeutic agent:
Much of the NAC sold in health food and similar food or drug stores today is packaged in containers that are not designed to protect NAC from air oxidation, and hence deliver increasingly more di-NAC as packages age, particularly when opened. I know of two companies, Zambon Pharma (Italy) and BioAdvantex (Canada and US) that currently produce and package NAC in ways to minimize oxidation. Zambon offers NAC as Fluimucil tablets, packaged in relatively small tubes that minimize NAC exposure to air; BioAdvantex offers NAC as PharmaNAC, effervescent “fizzy tabs” that readily dissolve in water, juice, or soda and are individually packaged in sealed foil packets to minimize exposure to air. Both companies have supplied NAC and placebo for clinical trials in our laboratory and elsewhere.2
This isn’t uncommon for products exposed to air to undergo oxidation. The same can happen for other antioxidant supplements, and is common for oils as well, hence the saying that “oils can spoil”. However, if the monomeric form of NAC is the one that’s most important then the dimeric form may reduce the amount of bioavailable NAC.
And speaking of “spoil” some of you may have noticed that your NAC supplements may smell… pretty rancid. Well, probably not on the same level as rotten garbage, but maybe something along the lines of an eggy smell. This smell may make it a bit difficult to take NAC supplements without getting disgusted.
That’s mostly due to the thiol (-SH) group from the L-cysteine. Thiols, and sulfur compounds in general, are known for their funk. Eggs are rich in sulfur compounds (they’re also a good source of natural L-cysteine) and so is garlic which is full of S-allyl cysteine compounds.
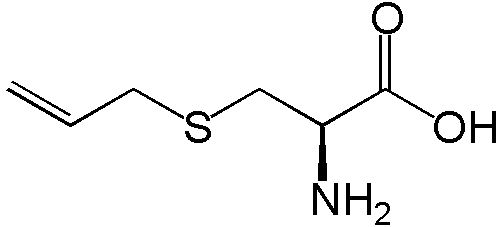
In fact, the gas used to heat our homes and stoves have sulfur compounds (usually hydrogen sulfide- SH2) added to give off that smell-otherwise we may not notice a gas leak inside of our homes.
Regardless, this foul taste and smell has made it difficult to give NAC to those who have overdosed on Acetaminophen, and is one of the reasons why NAC is generally given intravenously now in hospitals:
However, because NAC is readily oxidized to foul-tasting products, it can develop a foul smell that patients commonly find repulsive. I’ve lost count of the number of physicians who told me “horror stories” about having to convince people who have overdosed on APAP to swallow this life-saving brew.
Ironically, these observations could probably be used to gauge the quality of NAC supplements, such that higher quality supplements may have lover levels of oxidation and therefore a less foul odor and taste. This may not be the case in all circumstances, and the NAC dimers may not be all bad so this oxidation may not turn out to be too detrimental to supplementation.
For a concise summary of NAC pharmacokinetics refer to Box 1 of Tenório, et. al.
With that said, I do want to point to something that is generally used to dissuade at home supplementation, and that is toxicity.
Toxicity
Right now the FDA is reviewing NAC and searching for safety concerns with the drug. Many people may wonder if something offered as a supplement for decades may be considered relatively toxic. From all studies that I have looked at NAC has generally been considered to be safe and highly tolerable.
For example, overdose measures in animal models taken from Tenório, et. al. shows that- for most animals- the overdose measures for animals are over 1 gram/kg of bodyweight when given intravenously.
If we assume that the average 20 year old male is 80 kg, that means that 80 grams of NAC may need to be administered before serious cytotoxic effects may occur. Granted, we can’t extrapolate overdose measures in humans from animal models, but we may be correct in assuming that the overdose levels in humans are not going to be scant.
For example, one case report of an overdose incident of NAC involved a 23 year old woman who ingested several grams of Acetaminophen in order to commit suicide (Mahmoudi, et. al.5):
Four hours after taking 50 acetaminophen 325 mg tablets, a 23-year-old alert female patient weighing 65 kg presented to the emergency ward with weakness, lethargy, nausea, and dizziness. She had normal arterial blood gas and vital signs. Following administration of activated charcoal, in view of the toxic dose of acetaminophen ingested, treatment with NAC was started, with a 10 g loading dose of NAC 150 mg/kg given over 30 minutes, followed by a 9.5 g maintenance dose with 500 mL of 5% dextrose water administered over 20 hours.
Apparently, the nurse gave the wrong loading dose, and rather than administer 10g intravenously the patient was give 100g of NAC over 20 minutes, leading to severe adverse reactions and eventual death 12 days later:
Suspecting a drug allergy, the physician stopped administration of NAC, but further investigation revealed that a nurse had administered NAC 100 g (instead of 10 g) as the loading dose.
Remember that the bioavailability of NAC administered via IV is far higher than administered orally (due to the first pass effect), and therefore an extremely high dose of capsules would need to be taken to reach such a degree of toxicity. There’s also the matter of hepatotoxicity already occurring due to such a high dose of Acetaminophen, which may have also played a huge factor in the given outcome.
However, do not use this as validation to take as many NAC capsules as you want!
This is just merely an example of a human report of NAC overdose and many factors are at play that may cause adverse reactions to appear. So certainly do not use this as an example that you can take more- always consult the advice of medical professionals. Instead, this provides some insights and rebuts much of the claims that NAC may not be considered safe.
NAC does, however, have some contraindications, and taken with other medications may affect how those drugs are metabolized:
Therefore, if taking any of the above drugs it may be important to consult a physician before supplementing with NAC.
Pharmacodynamics
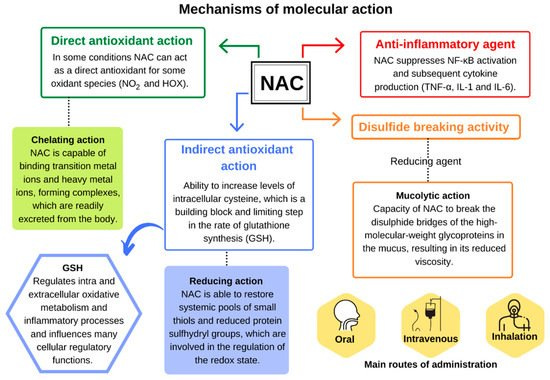
The mechanisms of action for NAC can generally be categorized into 3 main groups discussed briefly below but in greater detail further on:
Mucolytic: NAC has reductive capabilities, and therefore can reduce disulfide bridges found in various proteins. It’s argued that disulfide bridges are what lead to the mucous formation in COPD and illness. Therefore, removal of these disulfide bridges may “liquify” mucous and allow it to flow more freely.
Antioxidant: As a good electron donor, it’s suggested that NAC may operate in removing ROS and other free radicals. This may help alleviate oxidative stress, and therefore help reduce the burden caused by excessive oxidative damage on the body.
Restores Glutathione Levels: The most important role of NAC is to serve as a way of restoring glutathione levels after depletion. Glutathione is a tripeptide and serves as the biggest non-enzymatic antioxidant in the body. Glutathione is depleted when dealing with many issues such as Acetaminophen overdose or attenuating oxidative damage from an infection. Therefore, having the ability to restore Glutathione levels through NAC supplementation may help reduce duration of infection, reduce hangovers, and help recover from Acetaminophen overdose.
I. Mucolytic Agent
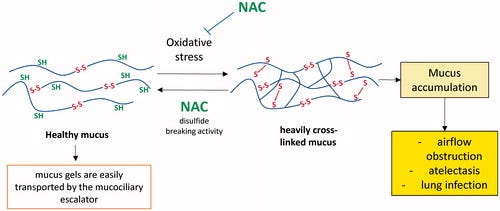
NAC was first investigated as a method of removing excess mucous. It’s assumed that some mucous may be inundated with heavy crosslinking between proteins through the formation of disulfide bridges between amino acid chains, possibly stemming from high levels of oxidation that aid in the oxidation of these thiol groups and causing the formation of disulfide bridges. It’s this heavy crosslinking of disulfide bridges that makes the mucus dense and difficult to remove.
Many bacteria also require the use of disulfide bridges in order to form biofilms, and therefore it is also assumed that NAC may also be able to target these agents as well. Essentially, many structures that require the use of a disulfide bridge may be targets of NAC.
NAC, through its thiol group, is suspected of acting as a nucleophile and targeting one of the sulfur’s from the mucous disulfide bridges and leading to a breakage, thus reducing the level of cross linking which makes the mucous more easy to remove (Aldini, et. al.6):
NAC is an efficient reducing agent of protein disulphides through the classic thiol-disulphide interchange mechanism [57]. An SN2 reaction mechanism is involved, whereby, in a single reaction step, the attacking NAC thiolate binds to the central sulphur of the disulphide and the leaving thiol (R″SH) is released via a trisulphide-like transition state structure. By considering this reaction model, the rate of the thiol-disulphide interchange reaction is strongly related to the nucleophilicity of the thiolate, and this explains the greater disulphide reducing ability of NAC compared with Cys and GSH [58], which reflects the order of S nucleophilicity of the thiols: NAC > GSH > Cys.
For organic chemistry buffs, the reaction supposedly follows the proceeding energy diagram (Nagy, P.7):
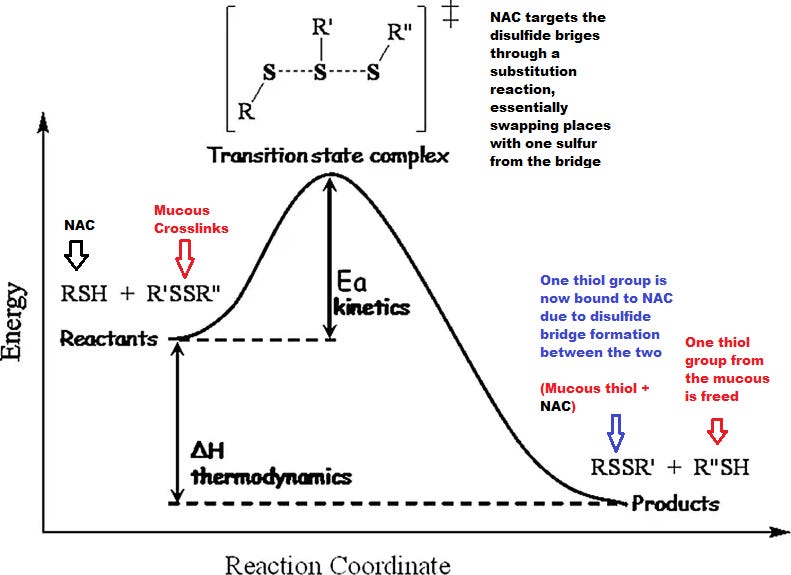
Interestingly, the targeting of disulfide bridges has been considered a possible method of targeting SARS-COV2, as many of the virus’ proteins bear critical disulfide residues (Wong, et. al.8, emphasis mine):
Envelope (E) and spike (S) proteins have a triple cysteine structural motif located directly after the E protein’s transmembrane domain (NH2- … L-Cys-A-Y-Cys-Cys-N … -COOH) and a similar motif located in the carboxy terminus of the S protein (NH2- … S-Cys-G-S-Cys-Cys-K … -COOH). The position, orientation, and composition of these two motifs may serve as a center for the structural link between the E and S proteins which is mediated by the formation of disulfide bonds between the corresponding cysteine residues.36 Previous studies have indicated that the entry of viral glycoprotein is affected by thiol-disulfide balance within the viral surface and the cell-surface of the host.37,38 Any perturbations in the thiol-disulfide interchange equilibrium would deter the entry of the virus into host cells.39,40 Cleavage of disulfide bridges by N-acetylcysteine disrupts the structural components of the interacting proteins, thereby impairing receptor binding affinity and infectivity.
This also includes disulfide bridges that serve as part of the structure for the ACEII receptor, with a suggestion that reduction of these disulfide bridges may alter the structure of these receptors and prevent the virus from binding:
N-acetylcysteine is a chemical reducer of disulfide bonds via its free sulfhydryl groups may interact with the extracellular disulfide bridges of angiotensin II receptor, alter its tertiary structure, and inhibit the binding of angiotensin II to its surface receptors (AT1a receptors) with subsequent attenuation of signal transduction and cell action. The AT1a receptors possess two sets of disulfide bridges at the extracellular domain of the receptors: C18-C274 and C101-C180. N-acetylcysteine can reduce the disulfide bonds in a dose-dependent manner,41,42 decreasing angiotensin II and increasing angiotensin 1–7 (a biologically active peptide exerting many opposing actions to angiotensin II), thus protecting against lung inflammation and fibrosis.43
Although this seems very promising, the targeting of host cells may not be very effective, and may actually alter important structural proteins since NAC would be relatively indiscriminate in its target. If that were to occur, NAC may cause damaging effects rather than therapeutic ones. NAC may also be considered a relatively weak reducing agent, with several studies showing these reducing properties taking place in vitro and over long periods.
This has been noted in the review from Pedre, et. al. It will be referenced a few times to provide contrast to some of the MOAs listed here.
Of note, the reviewers indicated that they could not find evidence of the actual mucolytic effects of NAC in the original clinical trials:
However, we could not find publications that provide direct evidence for protein disulfide reduction in the original clinical context, i.e. experiments that biochemically demonstrate the breaking of mucin disulfide bonds (and/or the formation of mixed mucin NAC disulfide conjugates) in patient samples obtained before and after in situ NAC inhalation/instillation. Instead, we encountered reports of recent clinical studies that have struggled to confirm the efficacy of inhaled NAC with respect to improvement of lung function or reduction in pulmonary exacerbations (Nash, Stephenson, Ratjen, & Tullis, 2009; Rogers, 2002; Tam, Nash, Ratjen, Tullis, & Stephenson, 2013).
This could be due to concentration differences in extracellular/in vitro studies, which may utilize higher levels of NAC than is given clinically. Studies that also looked at targeting cells tended to require high levels of NAC and long incubation periods:
How do these considerations relate to applications beyond lungs and pulmonary tissue? Direct reduction of protein disulfides on the cell surface seems to be plausible for in vitro cell culture experiments, because these often apply NAC at final concentrations in the mM range (5–20 mM) and over long incubation times. Such treatments have been found to lead to detectable protein disulfide reduction on the cell surface, although the efficiency seems to be low and the proportion of reduced disulfides is difficult to quantitate (Garant, Kole, Maksimova, & Bernier, 1999; Gelderman, Hultqvist, Holmberg, Olofsson, & Holmdahl, 2006; Kim, Rhim, Choi, & Kim, 2001; Laragione et al., 2003, Laragione et al., 2006).
Because of these issues there’s a few questions as to whether oral administration of NAC would have any mucolytic effects, especially since many concentrations from oral administration remain suboptimal for such effects:
In contrast, oral administration is reported to lead to NAC concentrations below 15 μM in the circulation (Holdiness, 1991). Thus, oral administration is the route least likely to support direct disulfide reduction in the circulation, or elsewhere in the body. It is therefore surprising that oral NAC, typically provided as effervescent tablets, is marketed in Europe as an expectorant, although it seems very unlikely that orally supplied NAC can reach the lung in sufficient amounts to actually cause disulfide reduction in the mucus.
So as a mucolytic agent and disulfide reducing agent there may be some doubts about how effective NAC would be. However, since NAC has several suspected MOAs it could be the culmination of effects that may provide the clinical benefits.
II. Antioxidant Properties
It has been suggested that NAC has the ability to act as an antioxidant by scavenging for free radicals and ROS such as hydrogen peroxide and hypochlorous acid, and reducing these agents into less toxic forms. Oxidative stress either through infection or other mechanisms may increase formation of radicals and oxygen species, so supplementation with NAC may seem tempting as a way to attenuate some of the damaging effects of excessive oxidative stress.
A review from De Flora, et. al.9 provides some insights:
Some major mechanisms responsible for the antioxidant/anti‐inflammatory properties of NAC are summarized in Figure Figure1.1. First of all, it has been established for a long time that NAC is a potent scavenger of ROS and especially of hypochlorous acid (HOCl) and •OH. 27 Inhibition by NAC of the ROS‐producing vascular NAD(P)H oxidases is relevant to prevention of hypertension and to pathological states associated with uncontrolled growth and inflammation, such as atherosclerosis. 28 The SH‐groups within the NAC molecule can also scavenge several reactive nitrogen species (RNS) that play a role in the oxidation of lipids, proteins, and DNA. 29 NAC potentiates the vasodilator and antiaggregatory effects of nitric oxide, which is a very important interaction clinically, and which has been shown to be valuable in the context of acute heart failure and acute myocardial ischemia and infarction. 30
However, Pedre, et. al. are far more critical of these assertions, as most of these claims of NAC may have been unfounded:
It is often claimed or assumed that NAC can protect cells against oxidative damage by directly scavenging ROS, in particular superoxide and peroxides. This notion seems to be the least supported and most problematic of the three classical narratives. It got off the ground in the 1970s and 1980s when protective effects of NAC in rats and isolated cells against cigarette smoke (assumed to contain or induce ROS) were noted (Moldéus, Berggren, & Grafström, 1985; Sprince, Parker, Smith, & Gonzales, 1975). Furthermore, cell culture experiments showed that NAC protected against exogenously applied oxidants, as generated from either natural (phagocyte co-culture) (Kharazmi, Nielsen, & Schiøtz, 1988; Simon & Suttorp, 1985) or artificial (bolus addition) sources (Junod, Jornot, & Grichting, 1987; Moldéus, Cotgreave, & Berggren, 1986). Although direct evidence was lacking, many studies attributed the observed cytoprotective effects to direct (i.e. uncatalyzed) oxidant scavenging by NAC.
This notion was widely accepted without direct evidence, probably because it has long been known that thiol groups are susceptible to oxidation in vitro and that their reaction with oxidants is highly favorable from the thermodynamic point of view.
Most of the issues come down to reaction kinetics. Much of that chemistry would be over the scope of this review, but the general assumption is that reaction constants for NAC are generally lower than other compounds and enzymes, meaning that NAC is not as effective at reduction as other cellular agents agents. Remember that there’s also the problem of bioavailability, such that NAC must be able to enter into cells and alleviate much of the oxidative stress taking place (Tenório, et. al, additional context provided):
Under physiological conditions, the reaction rate of NAC is usually lower when compared to other enzymatic and nonenzymatic antioxidants, as well as other substrates, which raises doubts about the relevance of its direct antioxidant capacity against RONS [reactive oxygen/nitrogen species], especially when other factors are considered as its endogenous concentration and location (intra- or extracellular) [3,11]. However, depending on its relative concentration compared to other thiols, it is possible that NAC has some direct antioxidant effect against some oxidative species, including •NO2 and hypohalous acids (HOX) [3].
So it appears that the actual antioxidant properties of NAC may be up for debate. Rather than act directly as an antioxidant, NAC may instead serve as a precursor for other compounds which have antioxidant properties. Therefore, NAC may not be an antioxidant per se10, but may help with reduction indirectly.
III. Glutathione Restoration
So far this review may have left a few of you a bit crestfallen. There’s quite a bit of controversy as to whether the prior mechanisms of action are actually how NAC operates.
But fear not, all hope is not lost yet! Our last topic will look at glutathione, and it’s here where NAC will actually truly shine. Out of all of the possible MOA’s, this is where there is substantial evidence in support for NAC.
Glutathione is a tripeptide created from the amino acids glutamic acid, cysteine, and glycine, and it’s considered one of the most important non-enzymatic antioxidants in our cells (Wrotek, et. al.11):
Glutathione (GSH) is the principal intracellular antioxidant buffer. It is predominately distributed in the cytosol (about 90%) and to a lesser extent in the subcellular organelles, such as the mitochondria, nucleus, and endoplasmic reticulum [67,68]. GSH is a ubiquitous, thiol-containing tripeptide produced by most mammalian cells. To synthesize glutathione, organisms require three amino acids: glutamic acid, cysteine, and glycine (Figure 2). The synthesis of GSH from its three amino acid precursors takes place in the cytosol. This process is catalyzed in all organisms by two enzymes: glutamate-cysteine ligase, regarded as the rate-limiting enzyme of the pathway, and glutathione synthase. The first step is controlled by negative feedback from its end product, GSH. When GSH is consumed and feedback inhibition is lost, the availability of cysteine as a precursor can become the limiting factor [67,69].
A schematic diagram of this reaction can be seen below:
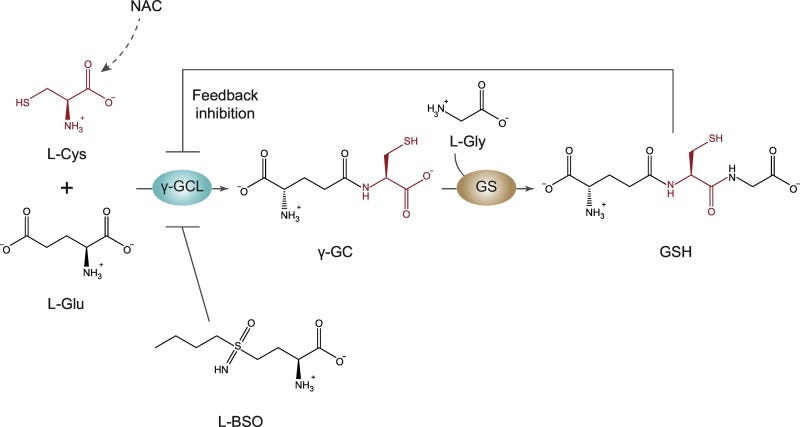
Essentially, NAC becomes deacetylated within the cytosol, and the increase in L-cysteine allows for the eventual formation of Glutathione (GSH). As GSH is one of the most important antioxidant agents, it makes sense why NAC may not act directly, but instead may act as a precursor for the formation of GSH, and it is through GSH that NAC likely serves as an antioxidant.
Now, you may have noticed that GSH depends upon two other amino acids (glutamic acid and glycine), so why supplement with NAC rather than the other amino acids? In this reaction L-cysteine is considered to be the limiting reagent, meaning that GSH formation depends predominately on intracellular levels of L-cysteine. There’s a few reasons for this. The most likely is that free L-cysteine concentrations are much lower than those of the other amino acids, and therefore less available L-cysteine means less GSH formation. Increasing intracellular L-cysteine levels are likely to overcome the rate-limiting step and allow for formation of GSH.
The significance of NAC as a way of replenishing GSH came about with studies examining Acetaminophen poisoning. Acetaminophen is metabolized predominately by liver enzymes, usually through the addition of sulfate and glucuronic acid (termed conjugation). These additional groups make Acetaminophen more water soluble and easier to excrete through the kidneys and urine. However, Acetaminophen may also be metabolized by P450 liver enzymes forming the molecule NAPQI. NAPQI may then be reduced by the addition of glutathione and then excreted from the body. However, at high Acetaminophen levels many of these agents may become depleted, leading to the buildup of NAPQI which may cause cytotoxic damage to the liver.
This effect can be explained further in the review from Attia, S. M.12
The major portion of paracetamol is conjugated with either sulfate or glucuronic acid to form water-soluble, readily excreted metabolites and only small amounts of the reactive intermediate, believed to be N-acetyl-p-benzoquinonimine (NAPQI), are formed by the cytochrome P450 enzymes (Figure 4). When therapeutic doses of paracetamol are ingested, the small amount of reactive intermediate forms is efficiently deactivated by conjugation with GSH. When large doses are ingested, however, the sulfate and glucuronide cofactors (PAPS and UDPGA) become depleted, resulting in more of the paracetamol being metabolized to the reactive intermediate.35–37 As long as GSH is available, most of the reactive intermediate can be detoxified. When the concentration of GSH in the liver also becomes depleted, however, covalent binding to sulfhydryl (-SH) groups of various cellular proteins increases, resulting in hepatic necrosis. If sufficiently large amounts of paracetamol are ingested, as in drug overdoses and suicide attempts, extensive liver damage and death may result.
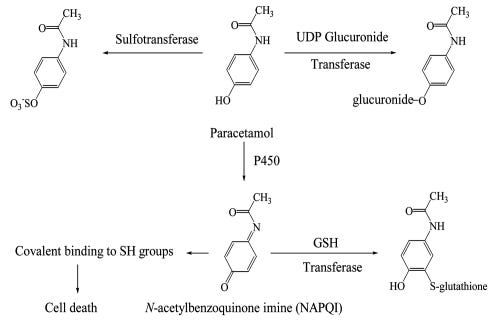
The addition of NAC here allows for repletion of GSH, recovering the removal of toxic NAPQI and alleviating the cytotoxic effects.
But GSH does far more than just serve as a protective agent against Acetaminophen poisoning. GSH can protect against oxidative damage from infections, attenuate inflammatory pathways, and providing benefits for chronic diseases that may lead to GSH depletion.
Unlike other mechanisms, it’s here where Pedre, et. al. agrees with these benefits of NAC via GSH recovery:
Following the paracetamol case, NAC has been shown to protect against other GSH-depleting hepato- and/or nephrotoxic xenobiotics, by the same mechanism, i.e. GSH replenishment. For example, NAC protected animals exposed to pesticides like fipronil, glyphosate, or paraquat (Abdel-Daim, Dessouki, Abdel-Rahman, Eltaysh, & Alkahtani, 2019; Turkmen et al., 2019; Yeh et al., 2006) or antibiotics like isoniazid, rifampicin, sulfasalazine or tetracycline (Attri et al., 2000; Farombi, Ugwuezunmba, Ezenwadu, Oyeyemi, & Ekor, 2008; Heidari et al., 2016). Overall, the idea of using NAC as a pro-drug of Cys to replenish GSH levels and exert a protective effect against GSH-depleting xenobiotics is well supported by the literature, in stark contrast to the disulfide reducing and oxidant scavenger narratives.
[…]
Apart from acute intoxication with xenobiotics, there are also chronic pathological conditions for which GSH depletion has been observed. Several studies claim that NAC elevated GSH levels and at the same time caused positive health effects. For example, NAC was reported to attenuate liver fibrosis in rats (Vendemiale et al., 2001; Y.-Y. Yang et al., 2008) and cystic fibrosis in humans (Tirouvanziam et al., 2006). Also, NAC was reported to protect rats against diabetic complications, decreasing damage of the sciatic nerve (Kamboj, Vasishta, & Sandhir, 2010) and of the liver (de Rosa et al., 2018). A clinical study reported that oral administration of NAC to HIV-infected subjects elevated whole blood and T cell GSH levels, and increased the two-year survival rate (De Rosa et al., 2000). In principle, it is plausible that the restoration of pathologically low GSH levels to normal levels can have positive effects beyond the protection against electrophiles and xenobiotics. GSH is a cofactor for glutaredoxins, hence involved in the catalytic reduction of disulfides (Deponte, 2013), and for glutathione peroxidases, hence involved in the catalytic reduction of peroxides (Brigelius-Flohé & Maiorino, 2013), including lipid peroxides (Brigelius-Flohé & Maiorino, 2013; Maiorino, Conrad, & Ursini, 2018). Indeed, several studies report that GSH depletion is associated with increased levels of the lipid peroxidation end product malondialdehyde, and that NAC can decrease these levels (Abdel-Daim et al., 2019; Attri et al., 2000; Farombi et al., 2008; Kamboj et al., 2010; Turkmen et al., 2019; Vendemiale et al., 2001; Y.-Y. Yang et al., 2008; Yeh et al., 2006). Moreover, the GSH redox potential is influenced by the total concentration of GSH (Meyer & Dick, 2010). Hence restoration of normal GSH levels may facilitate enzymatic disulfide reducing and oxidant scavenging activities that have been attributed to NAC itself.
In the context of COVID, it’s been alluded to that infection with SARS-COV2 may lead to depletion of GSH. This makes sense given the oxidative damage caused by viral infection.
Polonikov, A.13 provides a hypothesis for this GSH depletion from COVID:
Endogenous glutathione deficiency appears to be a crucial factor enhancing SARS-CoV-2-induced oxidative damage of the lung and, as a result, leads to serious manifestations, such as acute respiratory distress syndrome, multiorgan failure, and death in COVID-19 patients. When the antiviral activity of GSH is taken into account, individuals with glutathione deficiency seem to have a higher susceptibility for uncontrolled replication of SARS-CoV-2 virus and thereby suffer from an increasing viral load. The severity of clinical manifestations in COVID-19 patients is apparently determined by the degree of impaired redox homeostasis attributable to the deficiency of reduced glutathione and increased ROS production. This assumption can be supported by our findings. In particular, COVID-19 patients with moderate and severe illness had lower levels of glutathione, higher ROS levels, and greater redox status (ROS/GSH ratio) than COVID-19 patients with a mild illness. Long-term and severe manifestations of COVID-19 infection in one of our patients with marked glutathione deficiency suggest that the degree of glutathione decrease correlates negatively with viral replication rate and that an increasing viral load exacerbates oxidative damage of the lung. This finding suggests that the virus cannot actively replicate at higher levels of cellular glutathione, and therefore, milder clinical symptoms are observed with lower viral loads.
This may explain why NAC is being examined as a possible therapeutic agent against COVID. We’ll discuss the results of some of these clinical trials in another post.
There’s plenty more to say about the role that GSH plays (one more review to check out would be Forman, et. al.14), but note that GSH recovery is where NAC is likely to have the most profound effect, and much of the evidence in the literature points to this as being the most likely MOA of NAC.
The Benefits of NAC
As long as this post has been, this post is nowhere near exhaustive in its examination of NAC. Out of the possible 3 MOAs, it is through glutathione repletion that NAC is provides most of its therapeutic effects. Although the other two MOAs have been criticized here, it does not mean that these effects are not happening, but that more research may be needed to flesh out these effects.
We’ve also commented about NAC actually being a synthetic compound not normally found in nature. This may raise questions as to why it may not be easier to supplement with a natural compound such as L-cysteine rather than NAC, and we’ll save that explanation for the next post.
Regardless, NAC has had a very safe and tolerable profile. Reports of NAC overdose appear to be in response to heavy Acetaminophen consumption as seen in suicidal patients, and reports of NAC toxicity may only occur when severely high doses are provided intravenously and acutely.
This may raise questions as to what possible safety concerns the FDA may be researching. One would think that decades of over-the-counter use should provide robust evidence of severe toxic events, and yet much of the literature agrees with the notion that NAC is relatively safe, even if the effectiveness comes into question. Remember that drugs/supplements should attempt to satisfy the safe/effective paradigm, but when the effectiveness is brought into question the safeness should be considered.
Regardless, remember that it’s important to understand what a drug or supplement does before we decide to start using it. This information here is not intended to be medical advice, but as a way of informing people. There’s far too many issues in which people may act without being given the proper information, so hopefully posts such as these can provide better insights, and possibly change the way you think about what you put inside of your body. For those curious about reviews of other drugs, you can look for more posts under my Anthology Series Archive Tab.
Since this is becoming it’s own Anthology Series, I’m saving the review of clinical trials against SARS-COV2 for either the next post or a post sometime in the near future, so please be on the lookout for that!
If you enjoyed this post and other works please consider supporting me through a paid Substack subscription or through my Ko-fi. Any bit helps, and it encourages independent creators and journalists outside the mainstream.

You may sometimes see reference to NAC as N-acetyl L-cysteine or N-acetylcysteine. Structurally, they are both similar. However, N-acetyl L-cysteine indicates the enantiomeric form of the amino acid (L-enantiomer) while N-acetylcysteine does not make reference to the stereochemistry of the molecule. Just a bit of organic chemistry knowledge for those interested.
Pedre, B., Barayeu, U., Ezeriņa, D., & Dick, T. P. (2021). The mechanism of action of N-acetylcysteine (NAC): The emerging role of H2S and sulfane sulfur species. Pharmacology & therapeutics, 228, 107916. https://doi.org/10.1016/j.pharmthera.2021.107916
Tenório, M. C. dos S., Graciliano, N. G., Moura, F. A., Oliveira, A. C. M. de, & Goulart, M. O. F. (2021). N-Acetylcysteine (NAC): Impacts on Human Health. Antioxidants, 10(6), 967. MDPI AG. Retrieved from http://dx.doi.org/10.3390/antiox10060967
Herzenberg, L.A. (2019). History of N-Acetylcysteine. In: Frye, R., Berk, M. (eds) The Therapeutic Use of N-Acetylcysteine (NAC) in Medicine. Adis, Singapore. https://doi.org/10.1007/978-981-10-5311-5_1
Mahmoudi, G. A., Astaraki, P., Mohtashami, A. Z., & Ahadi, M. (2015). N-acetylcysteine overdose after acetaminophen poisoning. International medical case reports journal, 8, 65–69. https://doi.org/10.2147/IMCRJ.S74563
Aldini, G., Altomare, A., Baron, G., Vistoli, G., Carini, M., Borsani, L., & Sergio, F. (2018). N-Acetylcysteine as an antioxidant and disulphide breaking agent: the reasons why. Free radical research, 52(7), 751–762. https://doi.org/10.1080/10715762.2018.1468564
Nagy P. (2013). Kinetics and mechanisms of thiol-disulfide exchange covering direct substitution and thiol oxidation-mediated pathways. Antioxidants & redox signaling, 18(13), 1623–1641. https://doi.org/10.1089/ars.2012.4973
Wong, K. K., Lee, S., & Kua, K. P. (2021). N-Acetylcysteine as Adjuvant Therapy for COVID-19 - A Perspective on the Current State of the Evidence. Journal of inflammation research, 14, 2993–3013. https://doi.org/10.2147/JIR.S306849
De Flora, S., Balansky, R., & La Maestra, S. (2020). Rationale for the use of N-acetylcysteine in both prevention and adjuvant therapy of COVID-19. FASEB journal : official publication of the Federation of American Societies for Experimental Biology, 34(10), 13185–13193. https://doi.org/10.1096/fj.202001807
Pedre, et. al. argues that under the definition of antioxidant, NAC may not be considered an antioxidant if it does not act directly itself:
Although it may be difficult to give a generally binding definition for the term “antioxidant”, it is usually understood to mean a compound that directly and efficiently intercepts oxidants, thereby preventing the oxidation of other molecules. Under this definition, given the lack of evidence, NAC does not seem to qualify as an antioxidant in any relevant biological context. It may be argued that NAC still qualifies to be called an antioxidant when it acts indirectly, e.g. by being metabolized into a compound that is a bona fine antioxidant. While this position is debatable, we think that NAC should not be described as an antioxidant, as this propagates misunderstandings about the chemical nature of NAC.
Wrotek, S., Sobocińska, J., Kozłowski, H. M., Pawlikowska, M., Jędrzejewski, T., & Dzialuk, A. (2020). New Insights into the Role of Glutathione in the Mechanism of Fever. International journal of molecular sciences, 21(4), 1393. https://doi.org/10.3390/ijms21041393
Attia S. M. (2010). Deleterious effects of reactive metabolites. Oxidative medicine and cellular longevity, 3(4), 238–253. https://doi.org/10.4161/oxim.3.4.13246
Polonikov A. (2020). Endogenous Deficiency of Glutathione as the Most Likely Cause of Serious Manifestations and Death in COVID-19 Patients. ACS infectious diseases, 6(7), 1558–1562. https://doi.org/10.1021/acsinfecdis.0c00288
Forman, H. J., Zhang, H., & Rinna, A. (2009). Glutathione: overview of its protective roles, measurement, and biosynthesis. Molecular aspects of medicine, 30(1-2), 1–12. https://doi.org/10.1016/j.mam.2008.08.006
Great article! I only have one question- wouldn't it make more sense to just take glutathione? I'm currently taking that supplement...
Good article! This is very interesting to me, because I take 500mg N-Acetyl-L-Cysteine (Thorne) and 500mg glutathione reduced (Jarrow) as immune boosters, daily.