Adenoviral Vector Redux
A look at why blood clots may be so prevalent with adenoviral vector COVID vaccines.
Note: I have added corrections to the analysis of the Baker, et. al. paper. Please refer to the Viral Vector/PF4 section for corrections and additional figures added for clarity.
Out of the two main vaccine candidates available to the public the adenoviral vector based vaccines weren’t quite as popular. Maybe because its technology is not quite so new, and so it did not play on those same neurons that drive the need for impulsivity and novelty.
Nonetheless, millions of people were still provided these one-shot vaccine candidate, and a few months after widespread distribution clinicians began reporting on patients suffering from thrombosis (blood clots) a few days after receiving the adenovirus-based vaccine.
This led to quite a contentious predicament where the scope of the adenovirus-based vaccine’s use began to narrow, until last week where there was a near complete halt called on by the FDA to their use:
After conducting an updated analysis, evaluation and investigation of reported cases, the FDA has determined that the risk of thrombosis with thrombocytopenia syndrome (TTS), a syndrome of rare and potentially life-threatening blood clots in combination with low levels of blood platelets with onset of symptoms approximately one to two weeks following administration of the Janssen COVID-19 Vaccine, warrants limiting the authorized use of the vaccine.
When discussions of boosters began to arise a few months prior, I considered covering the Ad-based vaccines but decided against it. However, now appears to be a good time to go back and analyze these vaccines, and examine why blood clots may be so prevalent with these vaccines specifically.
Brief Introduction to Adenoviral-Vector Vaccine Development
Adenovirus-based vaccines (Ad-based vaccines) are an interesting concept. Instead of using inactivated virus particles or antigens from a specific pathogen, Ad-based vaccines utilize a separate virus- in this case an adenovirus- in order to deliver the necessary genetic material to host cells to produce the antigen in question (the spike protein).
Because a viral vector is being used to introduce the target gene there are some concerns about the activity of the virus’ genome and whether they may have the ability to reactivate. Several generations of Ad-based vaccines have attempted to solve this problem by snipping out a few genes to stunt the virus, and modern versions may remove all but the bare bones necessary for the basic functioning of the virus.1
The typical pathway for vaccine development utilizing Ad-vectors can be seen below (Hasanpourghadi, et. al.2):
Use of a viral vector has many benefits, as it may target specific cells based on the surface antigens of the virus, as well produce a high immunogenic response to viral vectors.3
However, it’s the latter that may cause a lot of problems. If the viral vector being used is one that people commonly experience, then there may be concerns that prior adaptive immunity to these viral vectors may eliminate the vectors before they can present the necessary antigen.
Prior Ad-vector studies utilized Ad5, a common human adenovirus which caused many issues due to prior antibody seroprevalence. One way to get around this prior immunity is to use recombinant viral vectors or animal-derived vectors. AstraZeneca made use of a chimpanzee adenovirus4 while J&J used a recombinant Ad26 viral vector, both of which tend not show high seroprevalence.
But it also creates another problem. Because these vaccines are based on viral vectors, there should be concerns that patients will produce an antibody response against the vector. That would mean that additional vaccination with these vectors via booster shots may be rendered ineffective due to an immune response to the first dose. This is the likely reason as to why a second dose was not considered5, and future booster considerations spaced out booster doses to much further into the future (3 months since 1st dose).
What’s the Deal with all these Blood Clots?
Before we look specifically at what may be causing these blood-clots with Ad-vector vaccines, let’s take a look at blood clots as they relate to a SARS-COV2 infection.
SARS-COV2 Infection and Thrombosis
Blood clot events have been widely reported with SARS-COV2 infection, and are likely to be related to increased platelet formation during an infection.
For instance, the cytokine storm experienced in more severe infections stimulate procoagulant events including platelet stimulation as well as thrombin production, among other various events.
A schematic representation can be seen below (Iba, T. & Levy, J. H.6):
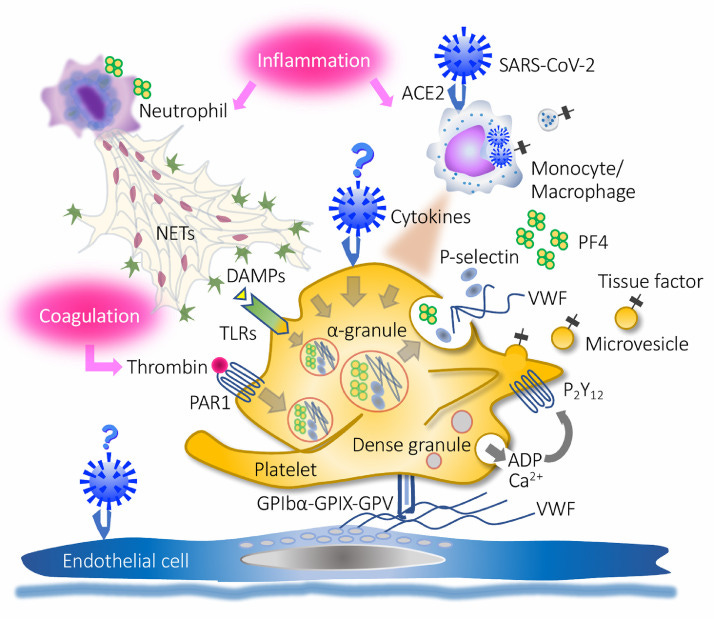
What’s important to note is that the spike protein itself has been indicated to be thrombotic, and therefore may play a role in blood clot formation itself. One study by Zhang, et. al.7 suggests that SARS-COV2 binding to ACEII receptors located on platelets may lead to platelet activation and clot formation. Another study by Zheng, et. al.8 indicated that SARS-COV2 spike proteins may bind to heparan sulfate and block binding of anticoagulants, thus contributing to further blood clotting.
So not only can the virus contribute to blood clotting, but the spike protein itself has the capabilities of leading to clot formation on its own- take a note of this since it will be important later on.
Vaccine-induced immune thrombotic thrombocytopenia (VITT)
Early reports of Ad-vector adverse reactions indicated thrombotic events as a serious factor. There have been several reports of thrombotic deaths occurring in vaccinees9 and is the likely reason for the FDA report sent out last week.
A few mechanisms have been proposed, but this review from Othman, et. al.10 provides a good rundown of some of these proposed mechanisms. An outline can be seen below:
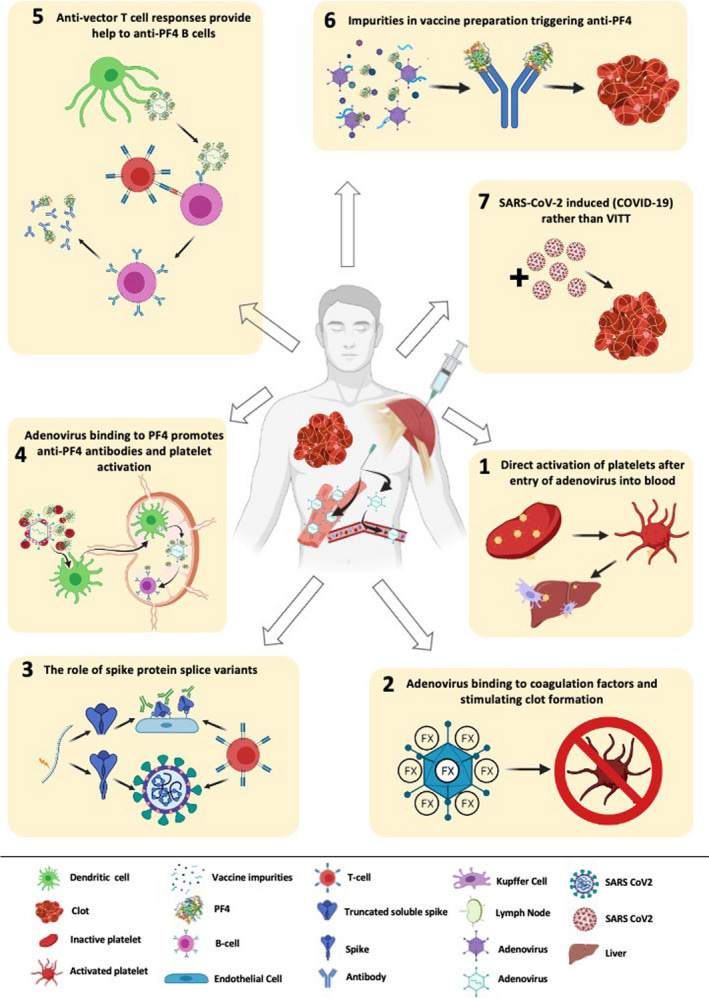
What’s interesting is that early reports of these thrombotic events did not appear similar to typical thrombosis, and instead manifested more like thrombotic events seen in patients given the anticoagulant drug heparin. This paradoxical disease, called Heparin-induced thrombocytopenia (HIT)11, is a disease in which patients experience low platelet count yet still present with thrombotic events. The results can be deadly- up to 20% of HIT events can result in fatality.
So why is it that patients who may not have been provided heparin but instead a vaccine still present with a diseased state similar to those provided heparin? This issue would require insights into heparin to further understand.
Heparin and HIT
Heparin12 is a naturally occurring polysaccharide (multiple sugars) comprised of glycosaminoglycans13 (GAG) and a negatively charged backbone. Glycosaminoglycans are involved in many intracellular and extracellular pathways and functions, and thus is vital to day-to-day cellular operations. Heparan (with an -a) is a good example of a commonly occurring GAG.
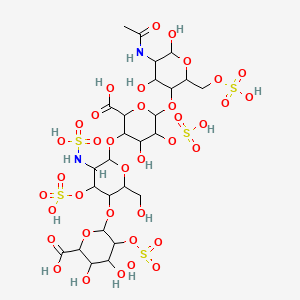
Heparin14 itself has been used extensively for many decades as an anticoagulant for the treatment and prevention of various diseases such as deep vein thrombosis (DVT), atrial fibrillation (AF), and pulmonary embolism (PE). Heparin is also typically administered before specific surgical procedures to prevent excessive blood coagulation.
Heparin has been indicated to bind to antithrombin and prevents the activation of thrombin and pro-coagulant events. It has also been indicated to be a possible anti-inflammatory as well as an antimicrobial.
Although heparin has been shown to be a widely effective drug, it does come with serious adverse reactions such as HIT. HIT appears to be caused specifically by an interaction between Heparin and a protein called Platelet Factor 4 (PF4).
PF4 are a class of chemokines released from activated platelets and are involved with suppressing the antithrombotic actions of agents found within the blood, such as Heparan lining the endothelial layer of blood vessels. Considering the structural overlap between Heparin and Heparan it makes sense that Heparin would be a target of PF4.
PF4- with its highly positive amino acid makeup- likely binds to the highly negative backbone of Heparin, but this binding leads to a conformational change of PF4 which exposes other parts of the PF4 protein. Unfortunately, these newly exposed regions are immunogenic, and IgG antibodies are produced to target this new PF4/Heparin complex.
This leads to an eventual positive feedback loop where IgG binding leads to immune and platelet activation with release of more PF4. If more Heparin is available, Heparin can then bind to more PF4 and elicit more IgG binding to these complexes.
Eventually this feedback loop leads to aggregation of these complexes and eventual clotting.
So how does all of this relate to VITT? Early measures of VITT indicated anti-PF4 antibodies, which suggest that a possible conformation change and exposure of PF4 immunogenic regions may be at play. In order for this to occur, PF4 must likely bind to a highly negative structure to expose these immunogenic regions.
Where are these highly negative regions located? It may not be a surprise, but there’s evidence that the viral vector itself may be the culprit.
Viral Vector/PF4 Interaction
If we point to the Figure from Othman, et. al. above we can see that one of the proposed mechanisms of VITT is a possible viral vector/PF4 interaction that stimulates anti PF4 antibody production and possible clot formation.
One proposal comes from a study by Baker et. al.15, in which researchers suggest a possible binding of PF4 to various regions of these Adenoviral vectors, including the ChAdOx1 and Ad26 vaccines.
Adenoviruses have an icosahedral (20 faces, like a 20-sided die) capsid structure studded with various fiber knob proteins.
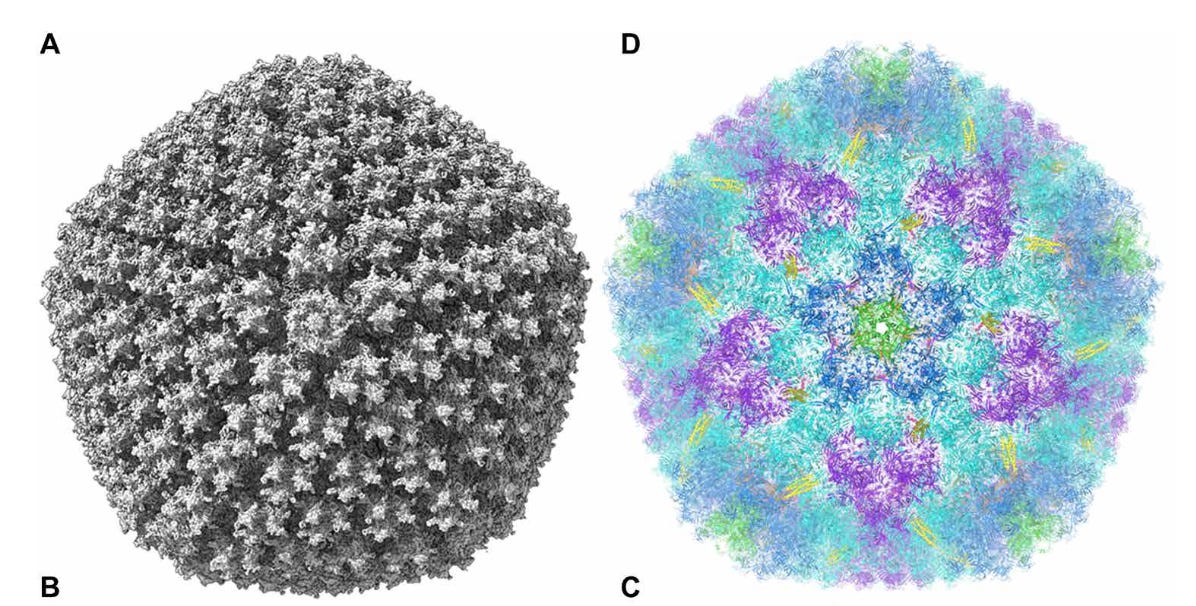
The researchers of this study, using computer analysis, was able to determine that most regions of the Adenovirus capsid has an electronegative potential and interacts with free-roaming PF4 proteins.
More importantly, regions between hexon proteins on the capsid’s surface were the most electronegative, and it’s these electronegative regions where PF4 likely binds.
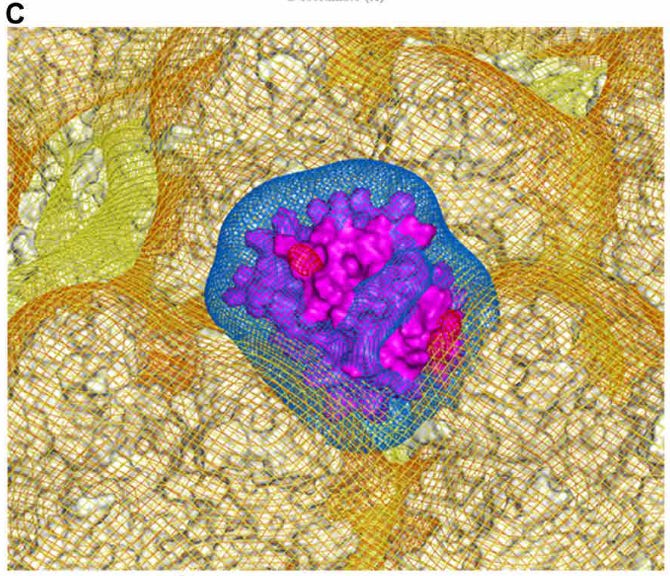
The researchers noted that this new Ad/PF4 complex may not elicit anti-PF4 antibodies themselves, but may activate a prior immune response instead:
A ChAdOx1/PF4 complex could induce anti-PF4 autoantibodies. In this potential mechanism, small quantities of ChAdOx1 enter the blood through minor capillary injuries caused by the intramuscular injection, as has previously been observed (35). A ChAdOx1/PF4 complex could then form (Figs. 5 to 7), either independently or in association with platelets (36), and travel to the lymphatic system, transported by monocytes. Alternatively, PF4 released at the site of injection may complex the vector and drain directly to the lymphatic system. These virus/PF4 complexes may stimulate preexisting anti- PF4 memory B cells to differentiate into plasma cells, secreting anti-PF4 antibodies, which generally takes 4 to 8 days (37).
What’s interesting is that the researchers noted that in vitro addition of heparin appears to inhibit Ad/PF4 complex formation, which further indicates that a viral vector/PF4 complex is likely involved in VITT.
Overall, this evidence helps to validate an actual mechanism of VITT, such that the viral vector itself may be contributing to clot formation.
Correction
As Brian of Unglossed pointed out, the electronegative regions between the hexon proteins of Ad26 are not as extensive as that of ChAdOx1, and therefore there needs to be considerations taken to account for possible differences in mechanisms. The researchers comment that there may be possible Ad26/PF4 complexes forming, but to the extent that it is occurring relative to ChAdOx1 should raise skepticism. Please keep in mind that the focus of this study was predominately targeted towards ChAdOx1.
For example, Figure 4 from the paper shows the following binding affinities. Note Ad26 (J&J) has lower binding affinity relative to the other tested adenoviral vectors.:
Figure 5C & D also indicates possibly higher electropositive interactions in Ad26. Please keep these values in mind.
Apologies for overlooking the study. And thanks for Brian Mowrey for pointing out these errors.
The Not-so-Innocent Vectors
The proposed mechanism above comes with a few caveats, but what it does suggest is that many parts of these vaccines may not just serve as innocent bystanders or carriers. I made this assertion with the LNPs, but upon further thought I’m leaning more towards the LNPs serving a possible carrier agents with their own forms of toxicity.
But more importantly, it could be the multifaceted insult on the body that may be leading to all of these adverse reactions. The inflammatory LNPs, the ER Stress caused by protein production, and cytotoxicity of the spike protein itself can all culminate to cause harmful side effects in vaccinees.
The adenoviral vector vaccines may suffer from the same problems. Not only may the manufactured spike proteins cause their own issues, but the viral vector itself may be responsible for their own thrombotic events. It’s a possible pairing of the two that may be further exacerbating the issues by providing two possible pathways of clot formation. What’s important is that thrombosis from Adenoviral vectors should present as different than thrombosis from spike proteins- clinicians should examine possible differential diagnosis to elucidate these differences.
Few studies take into consideration the entire vaccination process, and so we may not be aware of all of the possible insults that are occurring within the body.
What’s even more worrying is that no screening has been done to reduce risk of adverse reactions. The results of the Baker, et. al. study suggests that prior anti-PF4 antibodies may be reactivated, but we can’t discount the possibility that conformational changes to the PF4/Ad complex may elicit its own anti-PF4 antibody response.
Therefore, people with different comorbidities may respond differently to different COVID vaccines.
All of this indicates that not enough research has been done- there’s plenty more we need to know about these vaccines, and it’s likely that each component independently may cause their own issues, but that all of them together may worsen the risk of adverse reactions.
Hopefully more research will be done to see if the adenoviral vectors are part of the problem. I’m also likely to have left important ideas out due to email size so please ask questions below for further clarity or criticisms!
As to which path J&J or AstraZeneca took, I am unsure. If someone else is aware please let me know and I can update this article with that information.
Hasanpourghadi, M., Novikov, M., & Ertl, H. (2021). COVID-19 Vaccines Based on Adenovirus Vectors. Trends in biochemical sciences, 46(5), 429–430. https://doi.org/10.1016/j.tibs.2021.03.002
Kremer E. J. (2020). Pros and Cons of Adenovirus-Based SARS-CoV-2 Vaccines. Molecular therapy : the journal of the American Society of Gene Therapy, 28(11), 2303–2304. https://doi.org/10.1016/j.ymthe.2020.10.002
If anyone was curious, the name for AstraZeneca’s vaccine is derived from the viral vector being used: Chimpanzee Adenovirus Oxford [University] (who helped with the vaccine development), or ChAdOx1 for short.
Russia’s Sputnik V vaccine, which is an Ad-vector vaccine, uses a different vector for their booster dose (Ad5 and Ad26 were used I believe).
Iba, T., & Levy, J. H. (2022). The roles of platelets in COVID-19-associated coagulopathy and vaccine-induced immune thrombotic thrombocytopenia. Trends in cardiovascular medicine, 32(1), 1–9. https://doi.org/10.1016/j.tcm.2021.08.012
This is a really interesting article that provides a decent summary of thrombosis from both the virus as well as the vaccine, although I think the hypothesis for the negatively charged DNA backbone being the PF4 interactive agent does not appear likely. They provide another schematic outline for VITT as well:
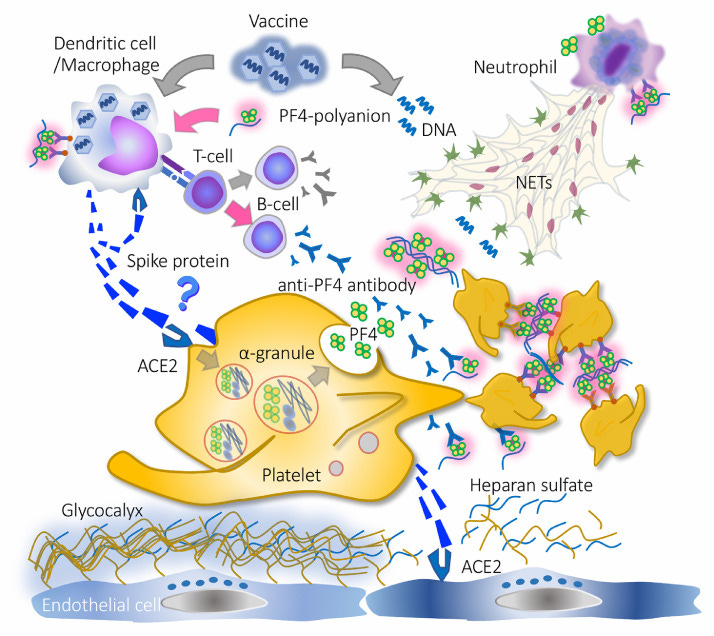
Zhang, S., Liu, Y., Wang, X., Yang, L., Li, H., Wang, Y., Liu, M., Zhao, X., Xie, Y., Yang, Y., Zhang, S., Fan, Z., Dong, J., Yuan, Z., Ding, Z., Zhang, Y., & Hu, L. (2020). SARS-CoV-2 binds platelet ACE2 to enhance thrombosis in COVID-19. Journal of hematology & oncology, 13(1), 120. https://doi.org/10.1186/s13045-020-00954-7
Zheng, Y., Zhao, J., Li, J., Guo, Z., Sheng, J., Ye, X., Jin, G., Wang, C., Chai, W., Yan, J., Liu, D., & Liang, X. (2021). SARS-CoV-2 spike protein causes blood coagulation and thrombosis by competitive binding to heparan sulfate. International journal of biological macromolecules, 193(Pt B), 1124–1129. https://doi.org/10.1016/j.ijbiomac.2021.10.112
Greinacher, A., Thiele, T., Warkentin, T. E., Weisser, K., Kyrle, P. A., & Eichinger, S. (2021). Thrombotic Thrombocytopenia after ChAdOx1 nCov-19 Vaccination. The New England journal of medicine, 384(22), 2092–2101. https://doi.org/10.1056/NEJMoa2104840
Long, B., Bridwell, R., & Gottlieb, M. (2021). Thrombosis with thrombocytopenia syndrome associated with COVID-19 vaccines. The American journal of emergency medicine, 49, 58–61. https://doi.org/10.1016/j.ajem.2021.05.054
Othman, M., Baker, A. T., Gupalo, E., Elsebaie, A., Bliss, C. M., Rondina, M. T., Lillicrap, D., & Parker, A. L. (2021). To clot or not to clot? Ad is the question-Insights on mechanisms related to vaccine-induced thrombotic thrombocytopenia. Journal of thrombosis and haemostasis : JTH, 19(11), 2845–2856. https://doi.org/10.1111/jth.15485
There are two types of HIT: Type I and Type II. Type I tends to be mild and occurs in a majority of HIT patients. Type II is the far more lethal form.
More information can be found here:
Oduah, E. I., Linhardt, R. J., & Sharfstein, S. T. (2016). Heparin: Past, Present, and Future. Pharmaceuticals (Basel, Switzerland), 9(3), 38. https://doi.org/10.3390/ph9030038
Glycosaminoglycans are disaccharide (2-sugar) molecules where one molecule is a sugar ring with an acidic group (aldonic acid) while another sugar contains an amine group (glucosamine).
Warnock LB, Huang D. Heparin. [Updated 2021 Jul 17]. In: StatPearls [Internet]. Treasure Island (FL): StatPearls Publishing; 2022 Jan-. Available from: https://www.ncbi.nlm.nih.gov/books/NBK538247/
Baker AT, Boyd RJ, Sarkar D, et al. ChAdOx1 interacts with CAR and PF4 with implications for thrombosis with thrombocytopenia syndrome. Sci Adv. 2021;7(49):eabl8213. doi:10.1126/sciadv.abl8213
Note that this was an in silico and in vitro study, so there are a lot of caveats to this study. This study also does not elucidate the actual direct mechanism, but provides a hypothetical for what these results may indicate. Further assessment is needed to validate or dispute the results of this study.
Please note: I have added further clarification and correction to the Baker, et. al. paper. I overlooked that the paper does indicate a large difference in binding affinity and electrostatic potential between the Ad26 (J&J) as well as ChAdOx1 (AstraZeneca), so please keep in mind that although the researchers argue that binding to Adenoviral vectors by PF4 may be occurring, the extent to which it is occurring in Ad26 viral vectors should be questioned. Apologies for overlooking this error.
Fantastic.
I have wanted to put a vector post together but my research was far less productive. There’s also very little info on what has been verified rather than assumed as far as the physical structure and in vivo behavior of the main two products.
AZ is two doses, spaced a weird amount of weeks apart. https://www.bmj.com/content/372/bmj.n421 refers to the normal 3 being adjusted to 12 during the UK rollout so I think 3 is the manufacturer's norm. E1 is knocked out, and it’s unclear to what degree this affects expression of other structural proteins. But presumably spike is still expressed, otherwise no antibodies. Except once again I’m reluctant to say this for sure, because what if there’s just leftover spike in the injection. Again, there’s no apparent lit from the manufacturers on this question.
I think JJ is same gene knocked out, let me check. Doorlesscarp also did a resource hub for the vectors here https://doorlesscarp953.substack.com/p/adenovirus-hepatitis-in-children